By Changwon Son, Ph.D., CSP, Assistant Professor, Department of Industrial, Manufacturing, and Systems Engineering, Texas Tech University, Lubbock, Texas, USA
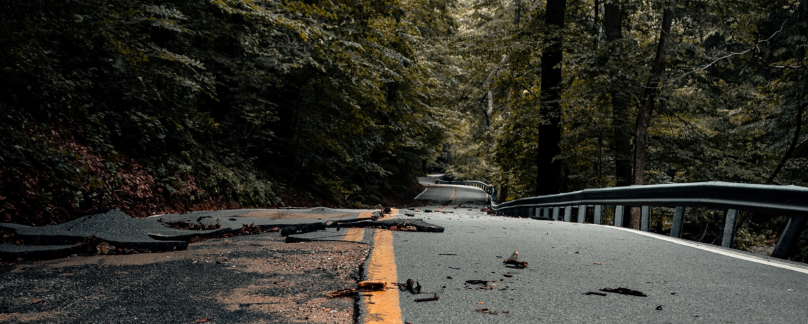
Communities in the world have experienced or witnessed increasing risks of disasters. Since 1980, the US has sustained over 300 climate-related disasters that caused overall economic losses of $1 billion or larger (inflation-adjusted, US NOAA, 2022). Not only that, but the frequency of disasters has also increased. In 1980, only an average of 2.9 large-scale disasters (with over $1 billion losses) had occurred annually. In the last three years (2019-2021), the average number of disasters has reached an average of 18.7 disasters per year. Economic losses incurred by weather-related disasters have also skyrocketed. Before Hurricane Katrina in 2005, the combined costs seldom exceeded $100 billion. Since 2005, the economic consequences of disasters have been increasing rapidly. One of the worst records in US history was marked in 2017 when Hurricanes Harvey, Irma, and Maria hit the US and Puerto Rico, resulting in a total loss of $346 billion. A similar tendency has been reported with respect to the whole world. According to a report published by the United Nations Office for Disaster Risk Reduction (UNDRR, 2018), the global economic losses incurred by disasters have steadily increased. Some major catastrophes are the earthquake in Sichuan, China (2008), the earthquake and tsunami in Fukushima, Japan (2011), or the disastrous hurricanes that hit the US (2017).
In addition to the increasing frequency and impact of disasters, recent years have seen a new challenge: compounding disasters. A compounding disaster initially meant cascading events–one incident leading to another incident. For example, the earthquake and resulting tsunami struck a nuclear power plant (NPP) in Fukushima, Japan. Consequently, the NPP lost the core cooling capacity of the reactors and eventually radioactive materials were released into the environment (Hasegawa et al., 2016). These days, compounding disasters represent multiple, concurrent incidents where one event makes it more difficult to respond to another, and vice versa (Potutan & Arakida, 2021). One common example of compounding disasters is the concurrence of the public pandemic (i.e., COVID-19) and other types of disasters such as wildfires, winter storms, droughts, hurricanes, volcano eruptions, and chemical explosion (e.g., ammonium nitrate explosion in Beirut, Lebanon in 2020). Such compounding events bring in unique challenges that were not seen often in the past. First, compounding events make it even more difficult to allocate resources that are already limited and oftentimes insufficient. Emergency medical service (EMS) providers, for instance, have to deal with patients from everyday incidents (e.g., a car crash) and those with COVID-19. Second, a response protocol for one disaster may not be compatible with another concurrent disaster. Wildfires that occurred in California, US caused thousands of evacuees who needed to be put in shelters. However, collocating the evacuees also raised concerns about increased risks of virus transmission. For another example, urban search and rescue (USAR) specialists were deployed to the city of Beirut in the aftermath of the massive chemical explosion. However, the USAR specialists had to follow a quarantine policy that prevented them from conducting immediate search and rescue operations. Third, compounding disasters make it more challenging to manage the information being fed by multiple sources and coordinate multiple agencies and organizations. Emergency managers have to process a constant influx of new information, including misinformation and rumors, from different sources and integrate them into meaningful intelligence. As multiple agencies operate concurrently, it may be challenging to prioritize response goals and objectives to be accomplished. For example, providing shelter to hurricane evacuees would be a priority to Red Cross; however, a public health department would prioritize individual evacuees’ risks of getting COVID-19 infection.
Experts anticipate that the current pandemic will not be over (Phillips, 2021). Moreover, there is no guarantee that a similar global pandemic will not occur in the future. This means responding to compounding disasters would be a new norm for many communities, if not all, in the world. Addressing compounding disasters is a multidisciplinary effort and there must be a role for resilience engineers to play in such an effort. As resilience engineering scholars claim (Hollnagel et al., 2011), lessons must be learned from the past and current responses to compounding events. Specifically, the focus of learning needs to be on adaptations that take place at different parts of an organization (e.g., sharp ends) and sources of success and failure in the responses. Lessons on adapted decisions, improvised actions, rebounds, and workarounds against the unique challenges from compounding disasters will fade away if they are not adequately observed, documented, and analyzed. Such lessons, if learned well, will help us better anticipate future compounding disasters–imagine a COVID-25 pandemic and other simultaneous events–and prepare for expected threats and even unexpected hazards. Then, the knowledge of our future threats and their impacts will let us monitor current preparedness (e.g., resources, plans, communication, decision-making policy) and identify gaps to be filled out.
Resilience engineering since its inception has contributed to addressing various socio-technical system problems such as healthcare resilience (Hollnagel et al.,2018). In the face of increasing disaster risks and unique compounding disasters, disaster resilience has become another goal of communities in the world, including us–resilience engineers.
References
NOAA National Centers for Environmental Information (NCEI) U.S. Billion-Dollar Weather and Climate Disasters (2022). https://www.ncdc.noaa.gov/billions/, DOI: 10.25921/stkw-7w73
United Nations Office for Disaster Risk Reduction (2018). Economic, Losses, Poverty & Disasters: 1998-2017. https://www.preventionweb.net/files/61119_credeconomiclosses.pdf
Hasegawa, A., Ohira, T., Maeda, M., Yasumura, S., & Tanigawa, K. (2016). Emergency responses and health consequences after the Fukushima accident; evacuation and relocation. Clinical Oncology, 28(4), 237-244.
Potutan, G., & Arakida, M. (2021). Evolving Disaster Response Practices during COVID-19 Pandemic. International journal of environmental research and public health, 18(6), 3137.
Phillips, N. (2021). The coronavirus is here to stay-here’s what that means. Nature, 590(7846), 382-384.
Hollnagel, E., Paries, J. Woods, D. D. & Wreathall, J. (2011). Resilience engineering in practice: A guidebook. Ashgate Publishing: Surrey, UK.
Hollnagel, E., Braithwaite, J., & Wears, R. L. (2018). Delivering resilient health care. Routledge: Newyork, NY.